The MRU and SRF Aligned (I)
With increasing accuracies of positioning and multi-beam systems, alignment of the motion reference unit (MRU) is becoming increasingly important. Although most (if not all) multi-beam surveyors use the patch-test to resolve the angular offsets between the multi-beam head and the MRU, this test does not give us the angular offsets between the MRU and the ship’s reference frame (SRF). In addition to this, any misalignment between the vertical rotation axis of the MRU and the SRF (yaw-angle) will introduce cross-talk between pitch and roll.<P>
The alignment of the threeaxes is also becoming increasingly important at shallower depths as we should try to position our vessel (or rather the transducer) within the dimensions of the footprint. This footprint will get smaller than 0.17 metres in water depths less than 20 metres for a 0.5° nadir beam.
Here in the Netherlands, most multi-beam vessels operate in waters shallower than 20 metres using real-time kinematic (RTK) for their 3D position. In a quest to achieve the highest possible data quality, the Dutch have developed methods to measure sensor offsets and to align the motion reference unit (MRU) with the ship’s reference frame (SRF). Over the years, this has resulted in a method that allows the alignment of the MRU with the SRF within 0.05° for pitch and roll and 0.3° for yaw.
As already mentioned, the patch test will not solve for the alignment angles between the SRF and MRU or multi-beam head. We may expect that the yaw angle found with the patch test is the sum of two misalignments: the misalignment between the gyro (which is often incorporated in the MRU) and SRF, and that between the multi-beam head and SRF. When we apply this yaw angle to the gyro or to the multi-beam head, we still have a misalignment between those two sensors on one side and the SRF on the other. This misalignment will introduce angular offsets to the lever arms between the antenna, MRU and multi-beam head and hence introduce errors in the X, Y and even Z positions of the latter.
The misalignment along the vertical axis of the MRU will also cause cross-talk between pitch and roll. Of all misalignments, this may be the hardest to solve. The MRU should be aligned in all directions with the SRF, which – although often chosen conveniently along the keel and waterline – seldom is reliably visible or not visible at all at the location where the MRU is mounted. The cross-stalk that concerns us most is induced roll by pitch, as this will have a major error contribution to the outer beams of the multi-beam swath. A yaw misalignment of 2° combined with a 5° pitch will induce a wobble on the outer beams of a 120° swath with an amplitude of 0.5% of the water depth. At 20 metres of water under the transducer, this is 10 centimetres (that is above and below the ‘real’ seabed, so a range of 20 centimetres in total). As the Dutch method allows for a measurement of the yaw misalignment angle to about 0.3°, the amplitude of the wobble will be reduced to about 3 centimetres under the same conditions.
The Dutch Method of Aligning the MRU
This method was developed by the Survey Department of the Directorate-General for Public Works and Water Management (Rijkswaterstaat) and comprises two separate procedures: the alignment of the vertical axis of the MRU with the SRF and the alignment of the pitch and roll angles, which will be discussed in Part II of this article. The best working order is to first determine the misalignment of the vertical axis and apply it to the MRU before calibrating the roll and pitch angles. In this way, pitch and roll output are corrected for any yaw misalignment and will improve calibration accuracy. In practice, however, both measurements are often combined due to time restrictions, leaving a small residual error that will be corrected for by the patch test. This method is justified as during those measurements pitch and roll of the SRF are commonly well within 1°, while the vertical axis of the MRU is usually aligned within 2°, leaving a residual error of less than 0.03°. This residual error can be corrected for mathematically or simply left to be solved in the patch test (although that would result in a 6-millimetre error with a 10-metre lever arm).
Both procedures require that the geometry measurement incorporates several fixed and well accessible points on board the survey vessel. Not only do these points serve as ‘geodetic base points’ for later geometry measurements, but they also serve as reference for the three-axis alignment of the MRU. Typically, the centre of at least four, but usually six or more bollards is used, all marked with a physical centre point.
Aligning the Vertical Axis of the MRU
Although calibrating the misalignment of the vertical axis is referred to as one procedure, there are three ways that are used for doing this:
1. physical alignment with a known direction within the SRF
2. alignment using the internal heading sensor
3. alignment using an external heading sensor.
1. Physical Alignment With a Known Direction Within the SRF
This method can be applied when the MRU is mounted against a known or well-defined reference within the SRF. A rib or bulkhead and sometimes the keel are commonly used for this. Of course, it should be possible to measure the direction of that reference within the SRF. Care should be taken that the reference chosen is straight between the measured points and that the distance between those points and their individual accuracies allows meeting the required angular accuracy. The keel is usually well defined outside the vessel (note that this sounds more obvious than it is), but sometimes far from straight as a result of all ribs and bulkheads welded to it. In general, the keel also curves up the bow and is therefore not always suitable as an accurate reference. The ribs and bulkheads themselves are usually quite straight, but difficult to see outside the vessel with enough accuracy.
When this method is used, it is usually done by transferring a reference from outside the vessel to inside by using well-defined features on the ship’s hull such as portholes or pipes for drainage or cooling water. From outside, these features can be measured with high accuracy, while on the inside the angle between the line through them and the rib or bulkhead can be determined using simple tape measures. For this, a thin wire is actually tightened between the measured points and tape measurements are taken from that wire to the bulkhead or rib while marking off the positions along the wire (or bulkhead or rib) to determine the distance over which they were taken. The distance along which this is done should again be long enough to meet the required accuracy. Presuming that the MRU axes are well aligned with its housing, we now can calculate the yaw angle.
2. Alignment Using the Internal Heading Sensor
As most Rijkswaterstaat multi-beam vessels are equipped with MRUs that incorporate an accurate heading sensor, this particular method is applied most often. Tests I performed while working at Rijkswaterstaat in the 1990s showed that the heading sensor was well aligned with the MRUs housing.
Ever since, the procedure has been quite straightforward. First, all existing offsets inside the MRU are set to zero, then a heading calibration is performed. As we need to find the misalignment between the MRU and SRF, the calibration has to be done in reference to the latter. One of the requirements of the geometry measurement mentioned above was that it had to incorporate several bollards. These bollards can now be used for the heading calibration. The heading calibration itself can be done at the quayside (see Figure 4) or by incorporating a known azimuth into the geometry measurement. The first method is most commonly used and allows the calibration of the heading in two opposite directions, which cancels out any directional errors. The quayside should be known in azimuth and straight enough not to affect accuracy, and at least 10 distance measurements (M1 and M2 in Figure 4) should be taken between it and the fixed points. Adding the distance measurements to the athwartships offsets of the bollards (O1 and O2) now gives the distances from the along axis of the SRF with the quay. Combined with the alongship distance between the bollards, we can now calculate the angle between the SRF and quay and thus the azimuth of the SRF. Although in the past I would read off the MRUs heading for every measurement to the quay, experience has taught me that one can log data during the measurements, average both the data and measurements, and use these averages to calculate the angular difference between the quay and SRF. The measurement is repeated with the vessel at opposite heading. The average heading offset found in this manner equals the yaw of the MRU and should be applied directly to it in order to allow it to correct for cross-talk. MRUs used on Rijkswaterstaat vessels calibrated in this way have a heading accuracy of 0.2° SEC (latitude) degrees, hence allowing calibration of their vertical axis to about 0.3° here in the Netherlands.
In some cases, two surveyed points (usually in or around the centreline) are used for this calibration instead of the bollards by measuring them simultaneously with two RTK receivers or, even better, by a RTK zero baseline. The quality of this reference can be checked by comparing the distance measured with RTK to the distance calculated from the geometry.
3. Alignment Using an External Heading Sensor
Not all MRUs have internal heading sensors, and so another procedure is required for these. As the second method works fine with MRUs that do have internal heading, we can make use of these properties when calibrating one that does not have them. For this, an MRU with internal heading is rented and physically aligned with the MRU that needs calibration. Now, the calibration is performed as described in the second method with the yaw angle as result.
The Influence of Roll
Calibrating at the quayside has a pitfall. The roll will influence the calibration as the used reference points onboard will probably not have the same height within the SRF and the higher point will be displaced athwartships more than the lower (see Figure 5). When subjected to roll, any pitch will either counteract or amplify this effect. The angular offsets found must thus be corrected using pitch, roll and the height difference between those points. Repeating the calibration in two directions will not necessarily cancel out this effect, as it depends on whether the roll was only caused by the persons that stood near the bollards (does cancel out) or the way the vessel was loaded (does not cancel out).
Results
Now that we know how to align the vertical axis of the MRU with the SRF, we would like to know how well the method works. In Figure 6, a summary is given of eight Rijkswaterstaat vessels calibrated in two directions as described above. For each instance, the difference between the two calibrations is given. Ideally, the difference should be zero, preferably less than 0.3°. The average calibration difference is 0.19° with a standard deviation (1?, 68%) of 0.15°. Although the figures do not tell us whether the MRU was properly aligned with the SRF, they do tell us that, in general, the repeatability is within the preferred values and we therefore may assume that alignment using this method was successful.
Acknowledgements
The Dutch calibration methods mentioned here and in Part II of this article were developed over the years by people like J.J. Knol, F. Maas, H. van der Marel, H. Murre, A.P.M. pieters, P. Kaslander, P. van Waalwijk and E.B. Wiegmann (see The History of the Dutch Method in the next issue of Hydro International). I wish to express my gratitude to them and all whose names are not listed. It is with thanks to all these people that we now know how to survey and calibrate a survey vessel properly. Many thanks to John Hughes Clarke for supplying me with images for this article and to Ad Pieters for filling in many historical details and commenting on the text. For the latter, I also wish to thank E.B. Wiegmann, S. Bicknese, R. Broekman and A. van Vliet of department DID-DSDH of Rijkswaterstaat.
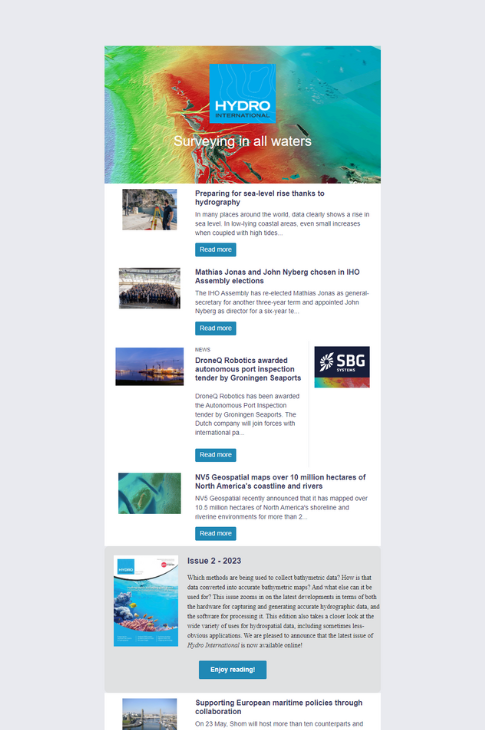
Value staying current with hydrography?
Stay on the map with our expertly curated newsletters.
We provide educational insights, industry updates, and inspiring stories from the world of hydrography to help you learn, grow, and navigate your field with confidence. Don't miss out - subscribe today and ensure you're always informed, educated, and inspired by the latest in hydrographic technology and research.
Choose your newsletter(s)