Airborne Lidar bathymetry in close up
Low-altitude surveying on the French Mediterranean coast
RIEGL tested the performance of its topobathymetric airborne laser scanning system in a transition zone context along the French Mediterranean coast. Initially planned as a UAV survey, tests were conducted using a fixed wing aircraft due to the restrictions placed on operations by the ongoing coronavirus pandemic. The results are encouraging, and the next step will be unmanned aerial vehicle (UAV or ‘drone’) operations in more challenging sea conditions.
Topobathymetric airborne laser scanning (a field of airborne laser bathymetry, or ALB) is known for its efficiency in seamlessly surveying hybrid landscapes. It is therefore used in hydrography and the maritime cartography of shallow water areas, and has the advantage of capturing topographic and bathymetric data in a single mission. These transition zones, which are difficult to cover by shipborne means or by land-based acquisition only, typically accommodate a large diversity of natural habitats and artificial structures, settlements or infrastructure. Consequently, they carry a high inherent risk due to terrain change, both offshore and onshore. These changes have to be monitored rigorously. The survey tasks are manifold, and include habitat preservation and protection, for example in areas that are exposed to flooding, land shrinkage or coastal erosion. Surveys are also used for infrastructure maintenance and to ensure coastal navigation safety. As a non-intrusive surveying method, ALB supports all of these tasks without impacting the environment and without putting surveyors at risk.
Lidar in bathymetry
The specific requirements and limitations of Lidar in the context of bathymetry have to be taken into account. After all, we are talking about light and water, and for all the beauty that these two evoke when they mingle, it means a meticulous balancing of the gain-and-loss ratio in Lidar technology. ALB systems are usually employed from manned aircraft at an operating altitude of > 1500ft (457m) AGL. They utilize green wavelength lasers, typically with a beam divergence of > 1mrad for reasons of eye safety, which is broader than that of the near infrared (IR) lasers used in purely topographic Lidar systems. The resulting laser footprint diameter on the ground surface is around 50cm. As a consequence, the planimetric resolution of the data is limited.
Turbidity further limits the usability of ALB. A coarse measure for turbidity is the Secchi depth, which refers to the depth that a white or black and white disc can be lowered into the water before it disappears to an observer on the surface. By using powerful lasers as well as large and sensitive receivers, it is possible to acquire data in multitudes of the Secchi depth. However, in turbid waters this can still mean quite poor penetration.
When the laser beam interacts with the water, it is not only scattered and attenuated due to absorption but its direction and velocity of propagation also change at the air/water interface due to refraction. Correcting for the effects of refraction requires accurate knowledge about the location where the laser beam hits the water, which can be determined by classifying the water surface points and generating a water surface model. These additional processing steps can be time-consuming and require experience on the part of the processing team.
Topobathymetric Lidar at low flying altitudes
Over the last decade, the use of unmanned aircrafts (miniature or midsized) as carrier platforms for cameras and all kinds of surveying equipment has been well established and has revolutionized the surveying sector. The availability of both UAS (unmanned aerial systems) and high-quality miniaturized sensors have enabled a new survey dimension in terms of perspective, flexibility and degree of detail. Based on this experience, recent research and industrial development have begun to focus on equally adapted sensor systems for hydrography.
While it is undisputed that bringing Lidar closer to the water surface restrains the swath width, and that the limited airspeed further reduces overall efficiency with respect to area coverage, this downscaling leads to a considerable gain in data quality concerning spatial resolution and potential depth performance. Expectations were especially high for complex, small area applications for the detailed mapping of riverine landscapes, which are underserved by high-altitude ALB.
RIEGL presented a first bathymetric Lidar profiler for UAS integration in 2016, followed by the first commercially available small-sized topobathymetric scanning system, the RIEGL VQ-840-G, in 2018. The core part of this system is a fully integrated compact airborne laser scanner for combined topographic and bathymetric surveying. The instrument can be equipped with an integrated and factory-calibrated IMU/GNSS system and with an integrated industrial camera. Compact and lightweight, with a weight of 12kg, it can be installed on various platforms, including UASs. The laser scanner comprises a frequency-doubled IR laser, emitting pulses with a roughly 1.5ns pulse duration at a wavelength of 532nm and at a PRR (pulse repetition rate) of 50–200kHz. At the receiver side, the incoming optical echo signals are converted into an electrical signal, amplified and digitized at a digitization rate of close to 2G samples/s. The VQ-840-G comes with special parametrization features allowing adaptation to the survey situation at hand. One option is to select the beam divergence between 1mrad and 6mrad to maintain a constant energy density on the ground and therefore balance eye-safe operation with spatial resolution. Also, the receiver’s iFOV (instantaneous field of view) can be chosen between 3mrad and 18mrad. For topographic measurements and very clear or shallow water, a lower setting is suitable, while for turbid water it is better to increase the receiver’s iFOV in order to collect a larger amount of light scattered by the water body.
The VQ-840-G employs a Palmer scanner generating an elliptical scan pattern on the ground. The laser beam consequently hits the water surface at an incidence angle with low variation. The onboard distance measurement is based on time-of-flight measurement through online waveform processing of the digitized echo signal. A real-time detection algorithm identifies potential targets within the stream of the digitized waveform and feeds the corresponding sampling values to the signal processing unit, which is capable of performing system response fitting (SRF) in real time at a rate of up to 2.5 million targets per second. These targets are represented by the basic attributes of range, amplitude, reflectance and pulse shape deviation and are saved to the storage device. Besides being fed to target detection and online waveform processing, the digitized echo waveforms can also be stored for subsequent offline full waveform analysis.
Testing the system on the French Mediterranean coast
After a series of successful test projects on various inland water bodies, with the VQ-840-G integrated both on manned helicopters and on two different models of electrical multirotor UAVs, an experiment by means of a manned fixed-wing aircraft was carried out in March 2021 on the French Mediterranean coast.
The choice of carrier platform was due to the current pandemic situation. Initially, the project had been planned as a UAV survey, but this had to be postponed throughout 2020. As personnel travel continued to be subject to restrictions, we found our crew better off in the air. And after all, it was a good opportunity to evaluate the performance of the system when operated from a manned aircraft. A Cessna T206H, OE-KRI, was used for the mission, at a mission flight altitude of 150m AGL and flying at 110kts, resulting in a point density of about 6-8 points/m² at the measurement rate of 50kHz we used for maximum performance. Of course, the flight dynamic of a manned fixed-wing aircraft is very different from the manoeuvring agility of a drone. And certainly, compared to typical drone flight parameters (e.g. 75m altitude; 20–30kts speed), the point density and performance was reduced, but this was offset by the flexibility and the possibility to cover a lot of ground in a short trip.
On 2 March 2021, the morning air was cool and calm as the Cessna T206 took off from Avignon-Provence airport for a first survey mission in the Camargue region west of Marseille. However, it had already started to rain in Montpellier further southwest and so another scheduled project there had to be cancelled in-flight. The OE-KRI crew decided to refuel in Avignon before continuing with the experiment in the rocky area of Cap Sicié and then continuing for more data acquisition towards the French-Italian border before returning to the home airfield in Austria in time. Ground handling was limited to the strictly necessary in order to minimize Covid-related risks for the crew.
Arriving at Cap Sicié shortly after 10:00 with an easterly force 4 wind, the sea state was slight. The waves reached 1–1.3m in significant height with a rather weak swell from the southeast.
Cap Sicié is a cliff off the bay of Toulon and La Seyne sur Mer in the French Provence area of Var. The cape is known because – in contrast to the sheltered bay area – the wind, in particular the Mistral and Levant, can be particularly strong. The highest point at 352m offers a panoramic view of Toulon Bay and, when the air is especially clear, much farther to the Côte d’Azur islands. To the east, an eye-catching twin rock formation, ‘Les Deux Frères’, served as a focus for our data acquisition.
Located just below the cape, Amphitria is a wastewater treatment plant, an astonishing architectural structure embedded smoothly into the steep cove. The building is exposed to both hazards from land and water, and therefore subject to continuous security measures, in order to ensure a safe working space for on-site personnel. The restricted accessibility due to topographic characteristics and protection of the natural habitat needed to be considered, serving as an example of the potential use of non-contact, yet close to surface, surveying methods.
Comparing the results with reference data
The test area (approx. 1152ha) was covered in 12 overlapping flight strips, resulting in a total of 60,864,774 single measurement points. For a point density check, only data from a single flight strip was taken into account. A first analysis of the Lidar data showed that the topographic parts provided reliable multi-echo coverage, thus well capturing terrain beneath vegetation. The seafloor in the measurement area is composed of sand, which provides good reflectance due to its colour yet causes water turbidity when disturbed.
In order to reference and validate the results, the data was compared with existing, publicly available ALB Lidar data obtained by SHOM (the French Naval Hydrographic and Oceanographic Service). In the national Litto3D programme, a digital altimetric reference model of the French coast for a littoral zone up to 10m isobath and up to 6 nautical miles offshore has been generated.
The acquired data was superposed onto the Litto3D data to obtain a visual impression of the two datasets. We were satisfied to find an excellent match of the point clouds both on dry ground and on the seafloor. While the Litto3D data extends to greater depth, the VQ-840-G’s data provides a more detailed view due to its higher spatial resolution. At the seafloor, the Litto3D data is specified to have 0.04 pts/m2, on dry ground this is 1 pt/m2. In the area we investigated, it is even slightly higher for both contexts. The VQ-840-G provided a resolution above and below the water surface of 7 pts/m2. These results might therefore perfectly complement wide-area survey data in regions of particular interest.
The brief field trip to the French coast provided insight into the VQ-840-G’s performance in a maritime context, operated from a manned aircraft. The results achieved are a motivation for taking the next challenge, which is to operate from a drone and in a higher sea state. A test series to further fine-tune the system for different survey applications is planned.
Acknowledgement
RIEGL would like to thank the local air traffic control service Base d'aéronautique navale de Hyères Le Palyvestre for flight coordination and Bateau-ecole de la Mediterrannée à Toulon for advice.
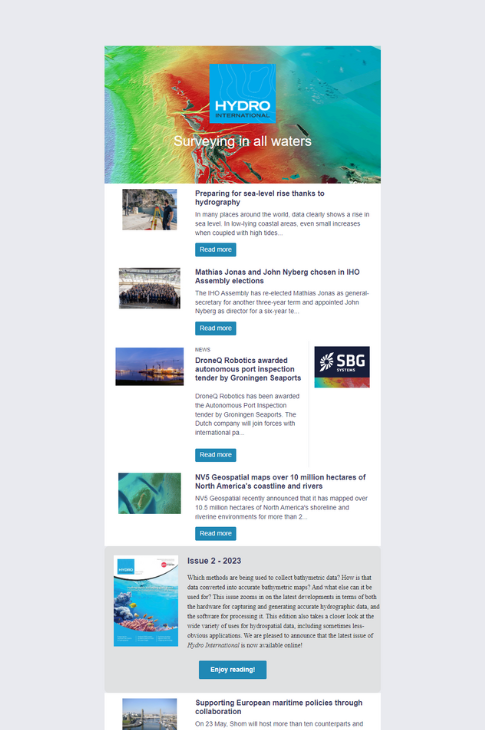
Value staying current with hydrography?
Stay on the map with our expertly curated newsletters.
We provide educational insights, industry updates, and inspiring stories from the world of hydrography to help you learn, grow, and navigate your field with confidence. Don't miss out - subscribe today and ensure you're always informed, educated, and inspired by the latest in hydrographic technology and research.
Choose your newsletter(s)